Jonathan Hood
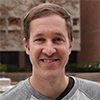
- Assistant Professor | Physical Chemistry
- Email: hoodjd@purdue.edu
- Phone: 765 494-5278
- Office: B155 BRWN
For Professor Jonathan Hood's individual Home Page click here
The goal of our experiment is to trap single ultracold molecules in optical tweezers with complete control of their internal and motional quantum states. Ultracold dipolar molecules offer a number of exciting new prospects for quantum technologies and for studying and controlling quantum chemistry. In our lab, we will assemble ultracold molecules from individual atoms using optical tweezers. The experiment starts by loading exactly two atoms into separate optical tweezers and then cooling them to the lowest possible motional state: the ground state of the tweezer harmonic oscillator. The two atoms are then adiabatically merged together, associated into a loosely-bound molecule, and then coherently transferred to a single deeply bound vibrational, rotational, and hyperfine state without motional heating. The optical tweezer array of molecules can then be reconfigured to for example bring two molecules together for a quantum gate, or to bring two molecules together for a reaction, or even to create a fully filled lattice of molecules to simulate a quantum spin model.
Quantum Computation and Simulation
A dipolar molecule has a permanent dipole resulting in long-range interactions between molecules — much stronger than the magnetic dipole interactions of neutral atoms. The long-lived ground rotational states of ultracold molecules are exciting new candidates for qubits in quantum computation or in quantum simulations.
Ultracold Quantum Chemistry
At ultracold temperatures, the de Broglie wavelength of a molecule is large compared to the interaction lengths scales, and quantum phenomena can dramatically change the interactions and reaction dynamics. For example, if two molecules are identical fermions, then the reaction can be suppressed due to the Pauli exclusion principle. Reaction dynamics can be dominated by quantum tunneling, or can be enhanced through Feshbach resonances with bound states. In addition, we see to control the reactions, effectively turning them on or off, by applying electric or magnetic fields, which orient the dipoles and greatly modifies the long-range potential.
Education
- B.S., University of Maryland, College Park, 2009
- Ph.D., California Institute of Technology, 2016
- Postdoctoral Fellow, Harvard University, 2019