Dr. Shelley Claridge
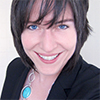
- Professor - Analytical Chemistry/Physical Chemistry/Materials Chemistry
- Email: sclaridg@purdue.edu
- Phone: 46070
- Office: 4150C BRWN
- Professor Shelley Claridge's individual homepage
A surprisingly broad range of problems in modern materials chemistry relates to structuring soft matter at scales well below 10 nm.
In this limit, an increasingly large fraction of functional groups lie at a boundary between two distinct chemical environments, which changes their behavior.
We examine the behavior of functional groups in dimensionally confined environments, with a particular interest in defining structural motifs that use these environments to advantage for the design of new materials. In order for these precisely structured environments to be useful, we also develop routes to simultaneously control structure at sub-10-nm to macroscopic scales, and to maximize stability toward common device processing conditions.
Functional group in a box.
Properties of matter change at the smallest scales.
How can we take advantage of these changes in designing soft materials for
energy conversion and computing near the molecular limit?
Inorganic
nanostructures exhibit confinement effects that change their physical
properties; often these are described in terms of a 'particle in a box' model
that quantifies changes in electronic behavior based on material dimension.
Similarly, the behavior of organic functional groups at interfaces differs from
those in bulk solutions, which becomes increasingly important as larger fractions of atoms are located at an interface. Because Angstrom-scale changes in position of a functional group relative to an interface change function, we leverage nanoscale characterization techniques including scanning probe microscopy to examine sub-nm-scale structure. Combining this information with characterization of physical properties at larger scales (such as contact angle measurements) enables us to derive new material design principles.
Bioinspired approaches to chemical confinement in
soft matter.
We are inspired by the chemistry of phospholipids, which
create well-defined 2D polar/nonpolar boundaries at the cell membrane periphery that enable a broad range of chemistry central to normal biological function. The chemical
diversity of lipids is vast -- many hundreds of structurally different
amphiphiles use precisely arranged sets of functional groups, as well as a
striking diversity of alkyl chains, to set the boundary structure. Transforming
these molecules into striped phases, in which the alkyl chains lie parallel to
the substrate, creates 1-nm-wide functional patterns of polar headgroups within
a predominantly nonpolar layer structure. We find that these highly confined
polar environments generate surprising new chemistry at the interface. Even in the striped phase structure, small amounts of water adsorbed to the polar headgroups plays a critical role in their function.
Maximizing processability, scalability, and function per mass. Designing materials with features at the 1-nm scale means building efficiently, with minimal waste. Simultaneously, to be useful in many applications, structures must be stable enough to survive reasonable device processing conditions, and scalable to areas of cm 2 or even m 2 . Striped phases are similar in thickness to a graphene sheet (nearly an order of magnitude thinner than a conventional standing phase monolayer), but embed desired functional groups in nm-wide arrays. At the same time, the noncovalent nature of the assembly raises significant questions related to robustness and utility for practical applications. Our group develops routes for achieving very long range order (>100 μm 2 ), improving processability (e.g. resistance toward desorption during solution processing) and for utilizing the functions embedded in the ultrathin molecular layer (e.g. in assembling nm-scale inorganic components on the template layer).
The questions we ask require us to design and synthesize new molecules with desired chemistry, assemble them, and characterize their function. Therefore, the research group is very interdisciplinary, drawing scientists with backgrounds ranging from materials characterization to organic and inorganic synthesis and theory. Students utilize facilities both within the laboratory and at the department's Analytical Instrumentation Center, and Purdue's Birck Nanotechnology Center and Life Sciences Microscopy Center. Techniques we use include scanning probe microscopies (including 2 AFMs and an STM as part of our lab), SEM, TEM, contact angle goniometry, XPS, fluorescence microscopy, CD, and PM-IRRAS. Students may also develop new instrumentation in conjunction with the Amy Instrumentation Facility, a unique resource in the Purdue Department of Chemistry.
Education
- B.S., Texas A&M University, Mathematics, Biochemistry, and Genetics, 1997
- Ph.D., University of California, Berkeley, Chemistry, 2008
- NIH Postdoctoral Fellow and Merkin Family Foundation Postdoctoral Fellow, Penn State/UCLA, 2013
Awards
- Schmidt Science Polymaths Award, 2022
- University Faculty Scholar, 2022
- Charles B. Murphy Award for Undergraduate Teaching, 2021
- ACS Nano iCANX Rising Star Lecturer, 2020
- Kohn Outstanding Alumni Lectureship, Penn State University, 2019
- Arthur Kelly Undergraduate Teaching Award, 2019
- DARPA Director's Fellowship, 2019
- DARPA Young Faculty Award, 2017
- JACS Emerging Investigator, 2017
- 3M Non-Tenured Faculty Award, 2016
- DuPont Young Professor Award, 2016
- Exceptional Early Career Award, Purdue University, 2016
- NSF CAREER Award, 2016
- Outstanding Undergraduate Teaching Award, College of Science, 2016
- Purdue Teaching Academy Fellow, 2016
- IUPAC Young Observer Fellow, 2015
- Teach for Tomorrow Fellow, 2015
- ACS PRF New Investigator Award, 2014
- Merkin Family Foundation Fellow, 2013
- UCLA Chancellor's Award for Postdoctoral Research, 2011
- UCLA Molecular Biology Institute Award for Postdoctoral Research, 2011
- NIH Postdoctoral Fellow, 2009
- UC Berkeley Outstanding Graduate Instructor Award, 2006
- UC Berkeley Everyday Heroes Award for Undergraduate Education, 2005